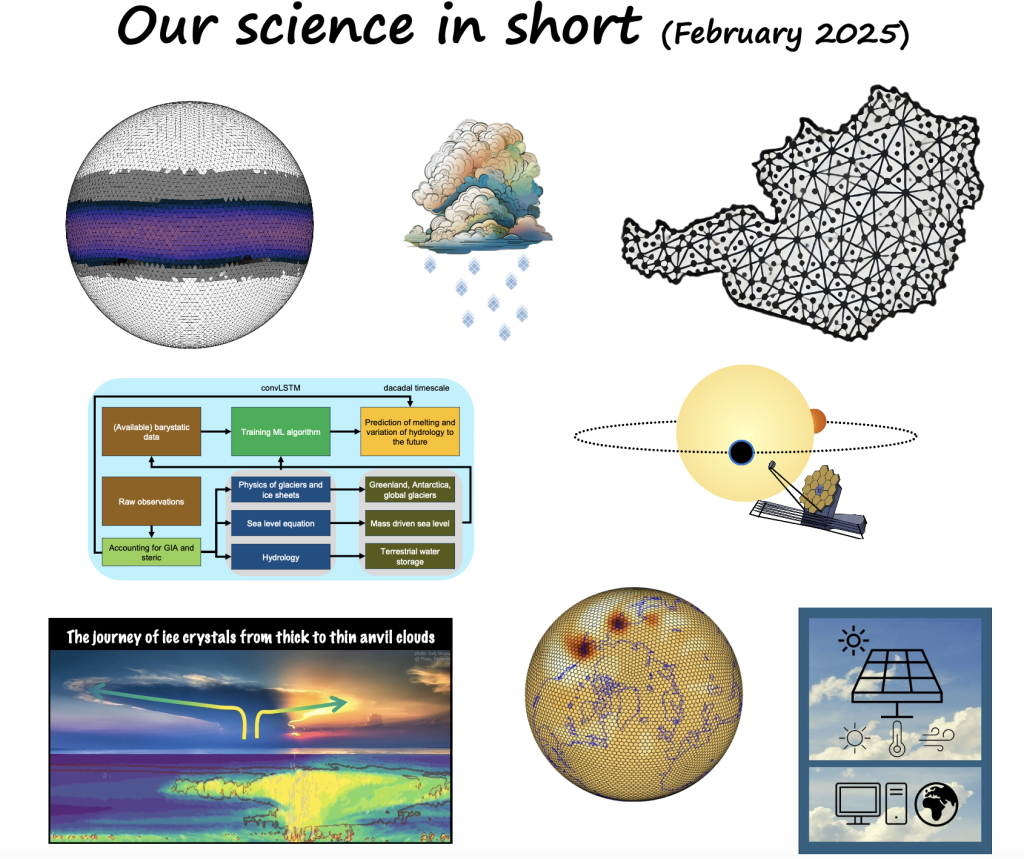
The video below shows a simulation of an extratropical cyclone with the ICON model at 2 km horizontal grid spacing and explicit deep convection, illustrating our use of atmosphere and climate models. Our research activities fall into four categories:
- Circulation of the atmosphere
- Clouds and radiation
- Snowball Earth and Exoplanets
- Computing climate at km-scale resolutions
Circulation of the atmosphere
The dynamics of the atmosphere shape regional climate and impact local weather. We are interested in the atmospheric circulation response to different drivers, including climate change, internal variability, and microphysical processes. We mainly focus on the northern hemisphere mid-latitudes and the tropics using a hierarchy of models ranging from idealized to fully coupled. In addition, we investigate how small-scale uncertainty (such as cloud processes) can feed up to the synoptic scale to influence the radiation budget and predictability.
In the past we have shown how clouds shape climate (e.g., the tropical rain belts and the extratropical storm tracks) via their coupling to the atmospheric circulation. We also research how small-scale microphysical uncertainties, like the interaction between cloud droplets and radiation, can control the radiative budget. Further we are interested in the interplay between the climate and weather times scales.
Key publications
- Keshtgar et al. (2023): Cloud-radiative impact on the dynamics and predictability of an idealized extratropical cyclone, Weather and Climate Dynamics https://doi.org/10.5194/wcd-4-115-2023
- Voigt et al. (2021): Clouds, radiation, and atmospheric circulation in the present-day climate and under climate change. WIREs Clim Change https://doi.org/10.1002/wcc.694
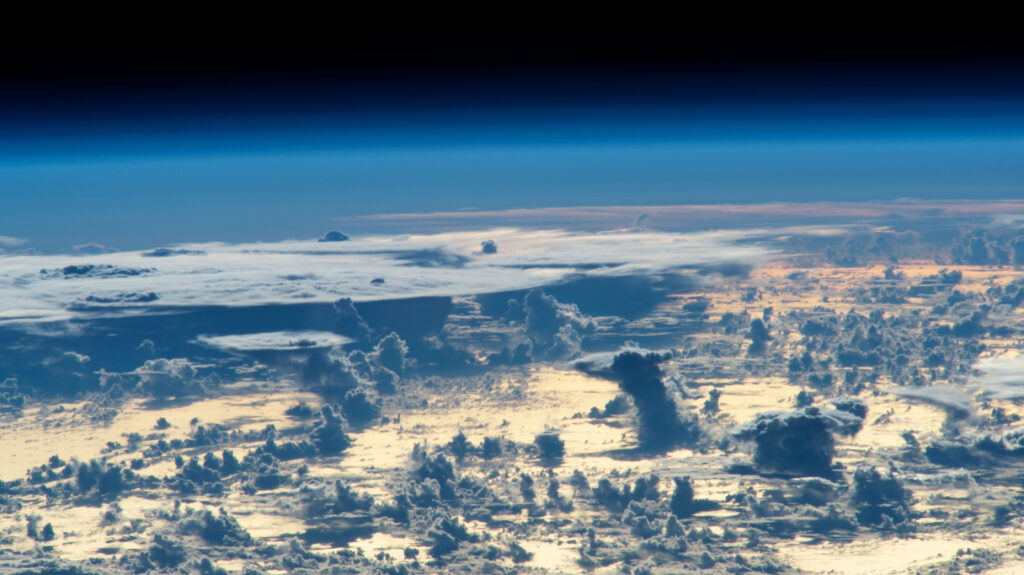
Clouds and radiation
Due to their interaction with radiation and their heating of the atmosphere, clouds are essential in shaping climate at both the global and regional scales. Thus, we believe that climate cannot be understood without also understanding clouds.
Our main interest lies on ice clouds since they play a large role in the radiative balance and through their heating in the upper atmosphere, they can influence the strength and shape the large-scale flow/circulation patterns. The way ice crystals form, grow, decay and interact with radiative fluxes is not well understood, which leads to uncertainties in representation of present day climate and in climate change projections. We try to address those uncertainties with the help of a hierarchy of climate models of increased complexity as better described under the climate computation tab.
Key publications
- Sullivan and Voigt (2021): Ice microphysical processes exert a strong control on the simulated radiative energy budget in the tropics. communications earth & environment https://doi.org/10.1038/s43247-021-00206-7
- Gasparini et al. (2024): Basic physics predicts stronger high cloud radiative heating with warming, Geophysical Research Letters, https://doi.org/10.1029/2024GL111228
Snowball Earth and Exoplanets
While surface ice is limited to high-latitude and high-altitude regions in today’s climate, there is ample evidence that this was not always the case. During the Neoproterozoic era 1-0.5 billion years ago, Earth experienced pan-glaciations in which all or almost all of Earth’s surface was covered in ice. We study the dynamics of these Snowball Earth states by means of hierarchies and ensembles of global climate models. A question of particular relevance to us is the existence of near-Snowball-Earth states that escape full glaciation and maintain a narrow strip of tropical ocean. Such states may explain the survival of life during the Neoproterozoic pan-glaciations and provide further insight into the habitability of exoplanets.
In fact, many aspects of the climate of a Snowball Earth might be more similar to that of extrasolar planets than modern Earth. The same fundamental physics that governs the climate of Earth allows one to study the climate of extrasolar planets. More than 5,000 exoplanets have been discovered to date, and the James Webb Space Telescope is expected to discover Earth-like exoplanets whose atmosphere contains water as a condensable component. We are applying our tools and understanding developed from studying the climate of modern Earth to such Earth-like exoplanets.
Key publications
- Braun et al. (2022): Ice-free tropical waterbelt for Snowball Earth events questioned by uncertain clouds, Nature Geoscience https://doi.org/10.1038/s41561-022-00950-1
- Hörner, J. and A. Voigt (2024): Sea-ice thermodynamics can determine waterbelt scenarios for Snowball Earth, Earth System Dynamics https://doi.org/10.5194/esd-15-215-2024.
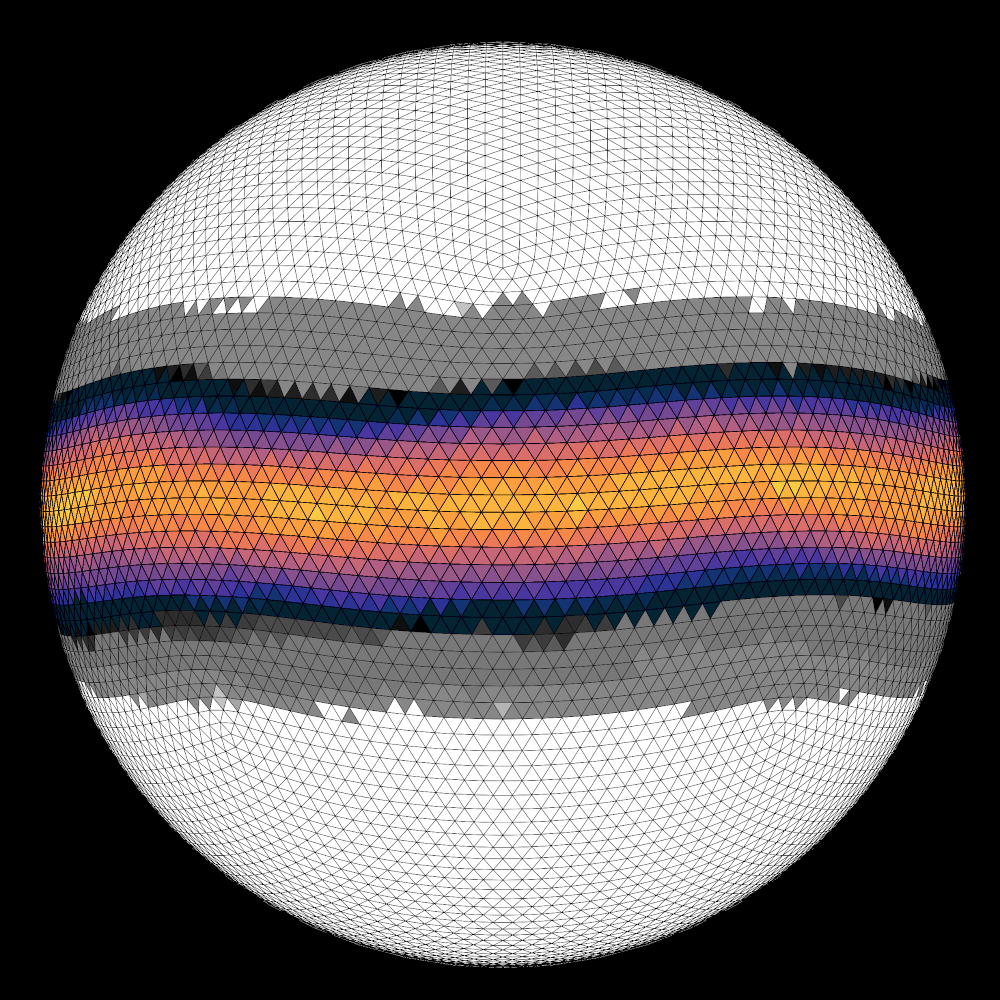
Computing climate at km-scale resolutions
Modeling climate and analying climate model data are at the core of what we do. Traditional global climate models with coarse horizontal grid spacings of around 100 km rely on empirical relationships to simulate convective systems and clouds. This has limited our understanding of the role of clouds and convection for weather and climate. New high-resolution models with grid spacings of 5 km or finer make it now possible to overcome this limitiation. These km-scale models can more accurately represent convective, cloud, and precipitation processes, offering a more physical and reliable representation of both global and regional climate. We use km-scale models to deepen the fundamental understanding of the role of clouds in the climate system and provide critical insights to inform decision-making in sectors such as infrastructure and energy systems.
Photovoltaics play a key role in decarbonizing the energy sector and therefore need to be resilient to climate change and extreme weather conditions. The main objective of “KlipPer” is to make the knowledge and data from climate modeling usable for the design and operation of a resilient photovoltaic infrastructure in Austria in a changing climate. We investigate future changes in photovoltaic yield over Austria and assess the added value of high temporal and spatial resolution climate data for PV yield modeling.
Within “HighResLearn“, we use machine learning to identify the added value of km-scale simulations. In particular, we estimate climatological model biases and climate change signals for the greater Alpine domain. Beside using ML methods for evaluating km-scale climate model output, we also develop data recipes that enable the climate community in Austria and beyond to easily access and process global km-scale model output.
Besides km-scale modeling, we continue to develop and run hierarchies and ensembles of climate models based on the low-resolution models ICON-ESM and CESM. We also benefit from community simulations from CMIP, SMILEs or Dyamond. The VSC Vienna Scientific Cluster is our main computational workhorse.
Key publications
- Brunner and Sippel (2023): Identifying climate models based on their daily output using machine learning, Env Data Sci, 2, E22 https://doi.org/10.1017/eds.2023.23
- Voigt et al. (2016): The tropical rain belts with an annual cycle and a continent model intercomparison project: TRACMIP. J Adv Model Earth Sy http://dx.doi.org/10.1002/2016MS000748
